Before we do anything with computers, let’s first create the universe.
OK, great. Now let’s try and populate it with stuff, by building up, not necessarily from the Big Bang onwards, but from matter as we know it today. To do this, let’s examine the building blocks that make up our world by looking at the best-ever theory of everything that we have so far. There are still quite a few mysteries regarding the universe still out there, such as dark matter, dark energy, and gravity. But this overview will grant us a solid foundation on which we can build a computer.
This best-ever (so-far) theory of everything is known as the Standard Model. It is a theoretical physics model of all quanta (more commonly denoted by the word particle) in the universe and how they relate to one another. A quantum is simply some tiny tiny quantized bit of matter. Imagine each quantum as being a billiard ball for now.
Every one of these billiard balls can have multiple properties. Those that are relevant to us right now are the first three of four properties (along with color change) of which are vital to understand: Mass, Electric Charge and Spin:
Mass is the fancy physics word for describing weight. Weight, technically, has a different meaning in physics than mass, but don’t worry about this too much: A 1-kilogram iron block “weighs” 1 kilogram anywhere on earth. But a 1-kilogram block in space might be weightless while still having the same mass of 1 kilogram.
Electric charge is a property that can take on 1 of 2 values: positive or negative. If you’ve ever played with two or more magnets, then you know that these magnets, arranged in a specific way, either repel or attract one another. Positive and negative charges are attracted to one another, while at the same time, when two of the same charges are brought together, makes them repel one another.
Spin is a little harder to grasp. Imagine spin simply being the “spin” of a ball, but at the quantum level. It is a sort of direction that the quantum spins in. Strangely, this number takes on values of either ½, -½, or multiples of these numbers (3/2, -2/2, etc.).
We’ve now described quanta in their most basic form. Let us now classify two types of quanta by understanding that different objects can behave in different ways. These behaviors are described by a statistic, which measures their overall behavior:
Fermions follow Fermi-Dirac statistics. These particles always have a half number of spin (½, 3/2, -½, etc.)
Bosons follow Bose-Einstein statistics. These particles always have a whole number of spin (1, 2, -1, 0, etc.)
This doesn’t tell us much about anything far, so let’s describe these two classes in more detail, starting with bosons. We can imagine bosons as being “interactive” particles that exist at the intersection between multiple fermions. The most famous boson is probably the Higgs Boson, which, via its field, gives everything mass. The other bosons are the photon, three types of bosons (W+, W- and Z) and eight types of gluons.
The photon is a quantum of light. Any light you see consists of billions of photons hitting the back of your eye and interacting with fermions there via the electromagnetic force. It is also the main way in which heat is dissipated from matter, meaning that if something is giving off heat, such as a kettle, it emits photons.
W+/- and Z bosons carry* the weak force. The weak force causes certain fermions to “transform” into other fermions. This force is also known as the weak force.
Gluons are special bosons that keep (certain) fermions “glued” together via the strong force, which gluons are carriers of.
This makes up the Bosons. Bosons are the particles/quanta that are used in order for fermions to interact with one another. Obviously, things are already getting difficult to track, so instead, so just remember: Gluons bind fermions, and photons exchange energy between fermions. Fermions are the main particles that make things up.
Now let’s look at fermions in more detail. Fermions can also be subdivided into groups, though thankfully only two: quarks and leptons. Let’s start with the leptons. Leptons are not affected by the strong force, which means that they tend to move more freely than quarks. In general, there is only one important lepton to remember out of the six that we know of: the electron.
The electron, with spin ½ or -½ and -1 charge, makes up one of the fundamental building blocks of not just computers, but of all perceivable reality. Photons for example, only (beyond a few very special cases) interact with electrons , which means that the existence of electrons is what allows light to be created in the first place. Additionally, whenever you think of electricity, you are likely thinking of billions of electrons, hence the name.
The other two leptons are the Tau and the Muon particle, which are highly unstable particles, each successively heavier, and transform into other particles via the weak force (i.e. the W and Z bosons). Simply remember that the electron has -1 charge for now.
Most of the most basic particles in the known universe.
Now to the quarks, which are the other types of fermion. Quarks make up the other ingredient of our perceivable universe. Quarks are all affected by the gluons which act as “glue” between the quarks, hence the name (naming is not always this nice, and will get a whole lot worse later on). Of the gluons, there are six different ones, only two of which are important to us at this stage:
Up & Down: These are the lightest two quarks, and have charges ⅔ and -⅓, respectively.
The other four quarks are known as the Charm & Strange quarks, and Top & Bottom quarks which are also successively heavier but also both highly unstable. Don’t worry about them.
Now the key point: Several quarks can be combined using gluons. Any combination of a multiple of quarks is known as a hadron. Most hadrons, as we will see, consist of 2 specific combinations of quarks (all other hadrons are mostly irrelevant to us (mesons, pentaquarks, etc.), as they are, as you might guess, also highly unstable). These two combinations of quarks are “two up, one down”, or “one up, two down”. If two up quarks and one down quark come together, then this composite particle is known as a proton with charge ⅔ + ⅔–⅓ = 1, hence the name (pro → positive, because charge is positive). This is the most abundant hadron in the known universe.
The second most abundant hadron in the universe is known as the neutron. This particle is created by combining two down quarks, and one up quark (along with the appropriate number of gluons), which gives us a new hadron of ⅔–⅓–⅓ = 0 charge, which as you can guess, is the reason why it’s called a neutron.
Now let’s create what has been named an atom. The characteristics of an atom are generally determined by the number of protons they contain. Usually, for whatever number of protons there are in an atom, there is a corresponding number of electrons to bring the combined charge to 0. Because electrons are leptons however, it means that they are not affected by the strong force. In other words, they interact with protons (and neutrons) differently than they do with one another. In other words, protons and neutrons interact with one another differently than protons interact with and electrons.
An atom without its electrons is known as a nucleus. The simplest nucleus is known as the hydrogen nucleus, which consists only of a single proton, along with some number of neutrons, usually zero. These hadrons are packed closely together due to the strong force. But Leptons are not affected by the strong force; they stay “outside” of the nucleus. The electron with its negative charge is attracted to the proton, so when an electron interacts with nuclei, they “bond” to transform the hydrogen nucleus to a hydrogen atom, whereby the electrons stay on the “outside” of the nucleus.
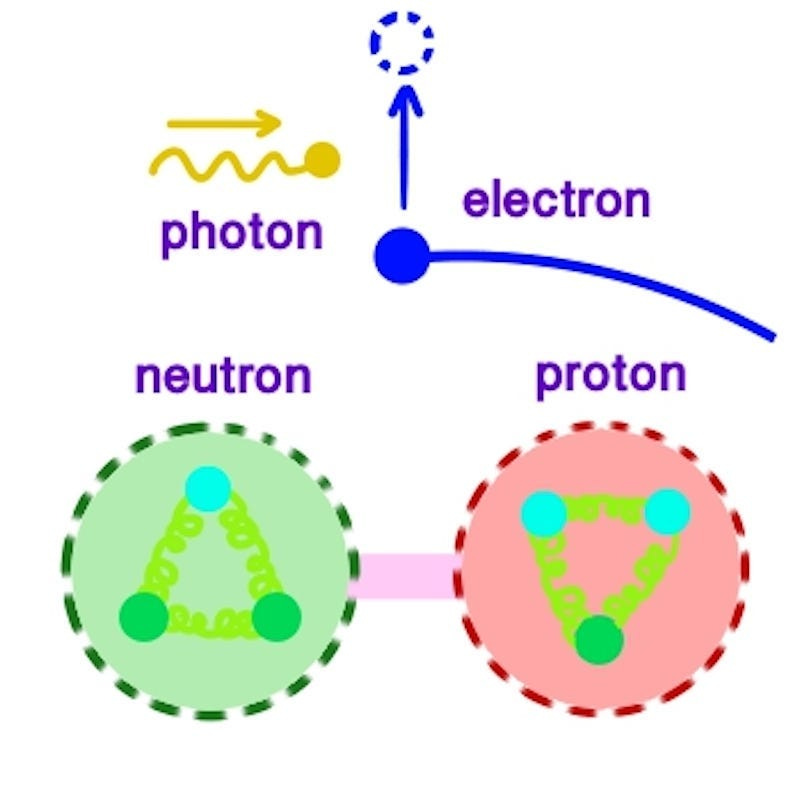
The fundamental building blocks of our known universe, and their interactions. The pink line is interaction between the quarks, via the strong force. Note also, that the neutron and proton could in theory produce a “heavy” hydrogen atom, known as a deuterium (1 proton & 1 neutron).
We can create more interesting atoms by having more protons in the nucleus. We know that two protons have a +1 charge, meaning that they would “repel” together. To stabilize atoms with multiple protons, you always need some number of neutrons. With every new proton, it is likely that they will attract an extra electron to make up for the imbalance of charge, but as we will see, this is not always the case. This, in essence, explains electricity at its most fundamental level: Electricity is created whenever there is a difference in charge between the two atoms.
All of the work to figure this out has been done in the 18th, 19th and early 20th century, which brought about several scientific revolutions, including but not limited to the study of chemistry, solid state physics and electromagnetism.
From here on out, we will need to go into more detail into these three disciplines to understand how we can manipulate the world, based on the knowledge that has been brought forth by these fields. Knowing that a computer consists of atoms is worthless without knowing the number of protons used in each of the atoms, how atoms interact in general, what sort of superstructures they create and also what the structure of the electrons in the atom are in the first place.
So let’s move on.
Footnotes
*As mentioned by one of my readers, the W-/+ Z bosons don’t “create” the weak force, and are not literally the weak force, but are instead the “carriers” thereof. Although this is doesn’t change the overall message, there is a subtle difference: “creating” something suggests that it didn’t exist before, which in our case is not true. “Being a carrier thereof” however, suggests that the force is still “in the universe”/existing, somehow, somewhere, which is more accurate. Additionally, these bosons have characteristics beyond just “being the weak force” (they have mass, spin, charge, etc.), hence why this linguistic difference likely exists in the first place.
Nice article. Some comments:
“Fermions follow Fermi-Dirac statistics. These particles always have a half number of spin (½, 2/2, -½, etc.)“ I assume you mean 3/2 instead of 2/2.
“Bosons follow Bose-Einstein statistics. These particles always have a whole number of spin (1, 2, -1, etc.)“ It’s worth including 0. Spin 0 particles include the Higgs, scalar and pseudoscalar mesons, and some larger composite atoms like He-4.
“three types of W+ and Z- bosons each“ There are three total weak bosons, not six (W+, W-, and Z).
“The photon is a quantum of light.” For consistency with the other two I’d mention that photons carry the electromagnetic force. Also, the thing you mention about heat transfer via radiation is true as long as you’re not touching the kettle; when you burn your hand most of the heat is transferred by conduction (hot atoms in the kettle directly jiggling atoms in your hand).
“W+/- and Z bosons create the electroweak force.” They’re the force carriers of the weak force. The electroweak force is a combination of weak and electromagnetic that only occurs at very high energy.
“Gluons are special bosons that keep fermions “glued” together via the strong force, of which gluons are the main carrier of.” Not just fermions — some bosons like the pion (or any meson) are bound together by the strong force. Anything that’s made of quarks (i.e. hadrons) is held together by the strong force. The strong force is even strong enough to hold multiple hadrons together in a nucleus.
“In general, there is only one important lepton to remember out of the three that we know of: the electron.” There are six leptons in total, electron/muon/tau and their respective neutrinos. I agree the electron is the only important one still.
“In other words, they interact with protons (and neutrons) differently than they do with one another.” Confusing wording since the pronoun ‘they’ refers to electrons the first time and nucleons the second time. I’d reverse the clause: “In other words, protons and neutrons interact with one another differently than they do with electrons.”